
"Just putting cells on a scaffold isn't enough. It's putting cells on a scaffold and giving them an electrical signal, and giving them a mechanical blood pressure, and then giving them oxygen... It's not just a heart in a jar, it's a heart in an artificial body: so it's simple in many ways, and it's unbelievably complicated."
- Doris Taylor, PhD, Director of Regenerative Medicine Research and Director of the Center for Cell and Organ Biotechnology, Texas Heart Institute, speaking in Nova Science Now: Can We Live Forever?
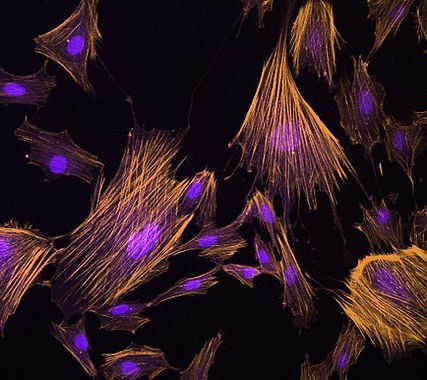
Microscope image of stained mesenchymal stem cells.
Option 3: Adult Mesenchymal Stem Cells
These cells can be found in whole bone marrrow, umbilical cord blood, fat, muscle, and in the pulp of baby teeth and the tooth bud of the third molar. Although they cannot make up an entire organ like embryonic stem cells can, they are able to make bone, fat, cartilage, pancreatic islet, and connective tissue cells. Because of their relatively non-controversial nature and relative ease of access, mesenchymal stem cells have become the cell type of choice for tissue engineering.
Option 2: Embryonic Stem Cells
"ESCs" are advantageous in tissue engineering because they can differentiate across a broad spectrum: the endoderm (lining of gut and other internal structures); the mesoderm (the middle part of the embryo from which the ESC was harvested) and the ectoderm (including the epidermis and the nerve tissue).
However, they are not without their own problems. Foremost, there is insufficient understanding about these cells, leading to incorrect differentiation and formation of tumors. In addition, embryonic stem cells are the subject of much heated ethical debate among political and religious parties.
What options are available?
Different areas of treatment typically have different cells that work the best; however, the main types of cells used for tissue engineering include differentiated primary cells, embryonic stem cells, and adult mesenchymal stem cells.
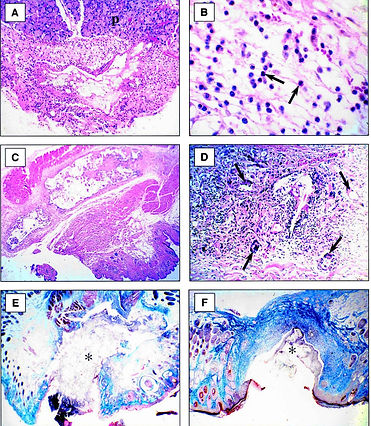
What is an extracellular matrix?
An extracellular matrix (ECM) is a non-cellular component of all tissues and organs. Made up of water, proteins, and polysaccharides, ECMs serve to provide structural support and therefore specific mechanical properties to the cells residing within them; in addition to this, they stimulate and trigger the cells to respond to their environment through bioactive cues. A key component of the ECM is that it must be flexible and able to respond to the dynamic processes of tissues (such as wound healing, homeostasis, or morphogenesis).
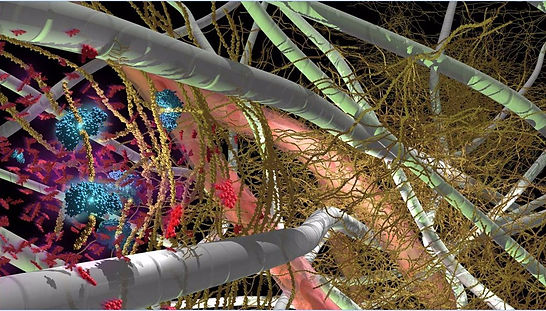
A depiction of the extracellular matrix at a microscopic level.
Scaffolds: Analogs to Extracellular Matrices
How can scaffolds emulate this?
In tissue engineering, the new tissue would ideally be in a scaffold identical to the target tissue it is replacing; however, this is not always possible to mimic exactly due to the multi-functionality, complexity, and dynamism of extracellular matrices. Instead, scaffolds can attempt to mimic the functions and features of the ECM - as outlined by B. P. Chan and K. W. Leong in their review "Scaffolding in Tissue Engineering:" architecture, cell/tissue compatibility, bioactivity, and mechanical properties.
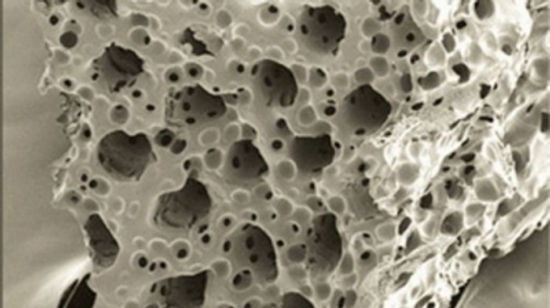
Architecture: the flexible material in this cardiac cell scaffold includes pores that allow movement of blood and nutrients; the larger holes allow the cardiac cells to fuse into their characteristic long chains.
1) Architecture
A scaffold needs to provide void volume (the volume of pores or spaces between particles) for the cells to form new tissues and vascularize (form blood vessels); it must have just enough pores to efficiently transport nutrients without becoming unstable. In addition, the biomaterials making up the scaffold should degrade at the same rate that the developing tissue is producing its own ECM so as to present a seamless transition and integration.
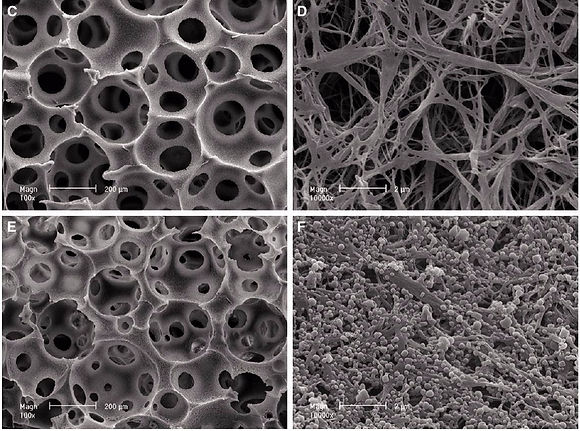
Scaffolds before (C and D) and after (E and F) the incorporation of the bioactive molecules (recombinant human bone morphogenetic protein-7).
4) Mechanical Properties
It has recently come to light that seeded cells depend on certain properties of the scaffold to differentiate properly. Cells such as muscle cells, epithelial cells, neurons, and fibroblasts all are able to sense the stiffness of the substrate on which they are seeded. No matter where in the body they were intended to be placed, if the stiffness is not correct, these cells will sense that the scaffold's stiffness is closer to that of a brain, muscle, or bone, and will differentiate into neurons, muscles, or bone tissues accordingly.
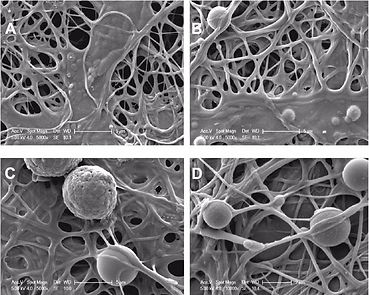
TE's Cellular Component: A Controversial Choice
Tissue Engineering: The Specifics

Tissue engineering (TE): "an interdisciplinary field which applies the principles of engineering and the life sciences toward the development of biological substitutes that restore, maintain, or improve tissue function" (Vacanti and Langer). Within this broad and sweeping field, there exist many variations on each of the ingredients and methods, but almost always key roles in the process are played by scaffolds, cells, and growth factors.
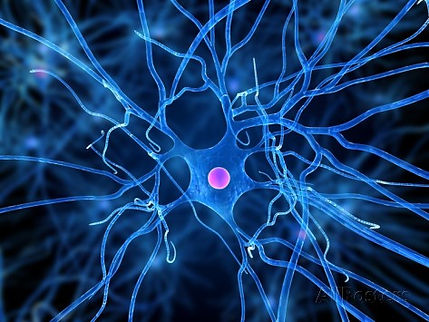
Innovative scaffolds using keratin from human hair to achieve non-toxic, thin fibers are shown here seeded with endothelial cells.
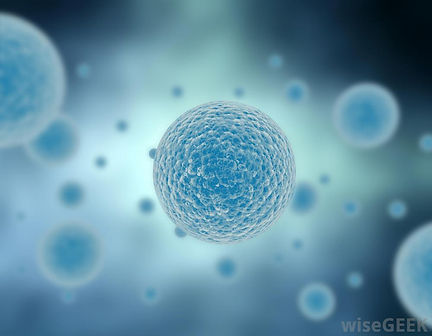
An artistic depiction of a cell.
Option 1: Differentiated Primary Cell
Most of the early successes in the field have come from cells taken from the patient; however these already-differentiated cells come with a host of problems. Cell collection is invasive, and cells can be in an unfit state due to disease or other damage; primary cells are difficult to expand in culture and out of the body tend to lose their capacity to form tissues.
A nerve cell is a dramatic example of how certain cells can differentiate to look much different than the original state.

A lack of compatibility can trigger an inflammatory response strong enough to destroy living cells
A diagram showing the diverse differentiation of an embryonic stem cell.
Regulatory Signals: Keeping Cells Alive
What is this third component of TE?
Dealing with living cells adds another layer of difficulty to tissue engineering. While the cells are outside of their organism - in vitro - they require growth factors, signalling molecules that instruct cells during their development.

Dr. Taylor's 'heart in an artificial body' demonstrating the regulatory signals necesssary to keeping beating hearts alive.
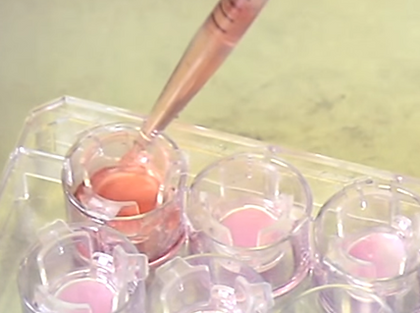
Cells receive growth medium after being seeded onto their scaffolds (shown in light pink)
2) Cell and Tissue Compatibility
Scaffolds need to allow cells to attach, grow, and differentiate during both in vitro (outside the body) and in vivo (inside the body); when the biomaterials used to compose the scaffolds are not compatible with either the engineered tissues or the host tissue cells, problems can arise. The adjacent diagram illustrates how an inflammatory response strong enough to destroy living host cells can result from a lack of compatibility.
3) Bioactivity
Scaffolds can interact with the cells in engineered tissues, regulating their activites. This can be done through delivering growth factors and anti-infection drugs loaded into the scaffold prior to cell seeding; these bioactive molecules can accelerate tissue regeneration. Interactions may also take the form of cell-adhesive ligands (a molecule/ion that bonds to a metal) to assist attachment, or even merely physical cues that influence the alignment and shape that cells will take. The adjacent image shows scaffolds before (C and D) and after (E and F) the incorporation of the bioactive molecules (recombinant human bone morphogenetic protein-7).